The research in my group focuses on numerical modeling of the atmospheric boundary layer, which we use to improve predictions for wind energy, air pollution dispersion, and cloud dynamics, among other applications. The analytical and computational methods that we develop help to quantify climate change mitigation strategies, air quality effects, and cloud representation and feedbacks in regional climate models.
Current projects include studies of wind turbine interactions with boundary layer dynamics over steep terrain, wildfire smoke modeling, urban dispersion modeling, landfill methane emissions modeling, and improved numerical techniques for turbulence, representation of topography, and grid nesting, among others.
The Environmental Fluid Mechanics and Hydrology at Berkeley page describes further research in our group.
Read this 2023 article from The New Yorker which talks about our recent wildfire smoke model research, Why It’s So Hard to Forecast Wildfire Smoke
Atmospheric dispersion studies – methane emissions
Methane (CH4) emissions from landfills represent a significant and under-characterized source of greenhouse gases, with implications for climate change, air quality, and regulatory compliance. Landfills are believed to be the third-largest source of anthropogenic methane emissions in the United States. Current measurement techniques used by the EPA are limited and rely on the wind blowing in the right direction to get downwind measurements with the tracer correlation method. We are using a set of nested atmospheric simulations to capture scalar plume transport from the landfill, given high resolution land surface and topography data. These simulations aim to identify best practices for emerging monitoring technology such as eddy covariance towers, drones, and satellites. This will help the EPA develop standards for landfill emissions, which are currently self-reported with large inherent error bars. This work is in collaboration with Paul Imhoff at the University of Delaware and with Ramin Yazdani at UC Davis. See Delkash et al. 2022, Taylor et al. 2018, 2016. Video: Parker Yang
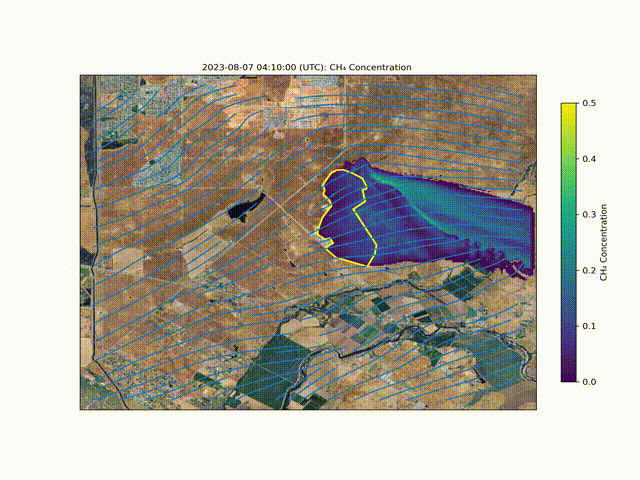
Neighborhood-scale air quality
Neighborhood-scale air pollution hotspots have recently been identified through detailed field campaigns, including the 100×100 Black Carbon Experiment which took place in West Oakland, CA, in 2017. We performed high-resolution nested atmospheric simulations with a Bayesian inversion framework to estimate source apportionment at the hyper-local scale for a neighborhood in West Oakland. Data from 12 black carbon sensors are used to perform source inversion using a Markov Chain Monte Carlo approach, which provides a probability distribution for each of the 11 source strengths. With this probabilistic analysis, it is possible to determine areas in the neighborhood with particularly high black carbon concentrations. See Chen et al. 2024.

The Terra Incognita – or the Gray Zone – in atmospheric modeling
One of the current hot (and challenging) issues for turbulence simulations is the so-called gray zone or terra incognita. When the grid resolution is too coarse to resolve any turbulence, a full turbulence parameterization is used. When the grid resolution is fine enough, explicit turbulent flow structures can be simulated, as in LES. A problem occurs when the grid resolution is only fine enough to partially resolve the turbulent flow (in the atmosphere this happens for a grid spacing of around 1 km) – this is the region called the gray zone. Numerical weather prediction models are increasingly crossing into the gray zone, because of the trend for models to move to higher resolution. Simulations operating in the gray zone in the atmospheric boundary layer can produce ‘false’ convection, with unphysical convective cells. Simulations at higher resolution than previous forecasts can thus counter-intuitively produce more erroneous results. These findings are critical for models crossing into the gray zone (e.g. as being proposed by several national weather agencies across the globe) and will require new model configurations to help counteract the erroneous model behavior. See Efstathiou et al. 2024, Chow et al. 2019, Zhou et al. 2014.
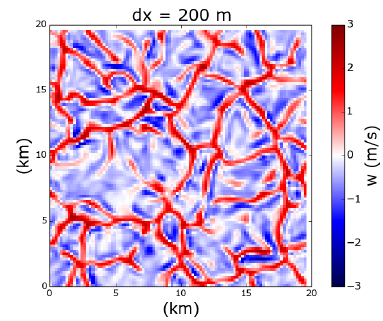
Wind energy simulations over complex terrain
Stable stratification has been the object of numerous field and modeling studies, but the presence of intermittent turbulence continues to challenge the abilities of fine-scale atmospheric simulations for wind energy. By leveraging the extensive data from the NSF-funded study of the double-ridge Perdigão valley of Portugal in May-June 2017 and the DOE-funded AWAKEN field campaign in the great plains in 2024, we study the fundamental atmospheric flow dynamics in complex terrain using a suite of observational and modeling tools. Our focus is on mechanisms controlling turbulence intermittency in stable boundary layers in complex terrain. See Wise et al. 2022 and 2025.
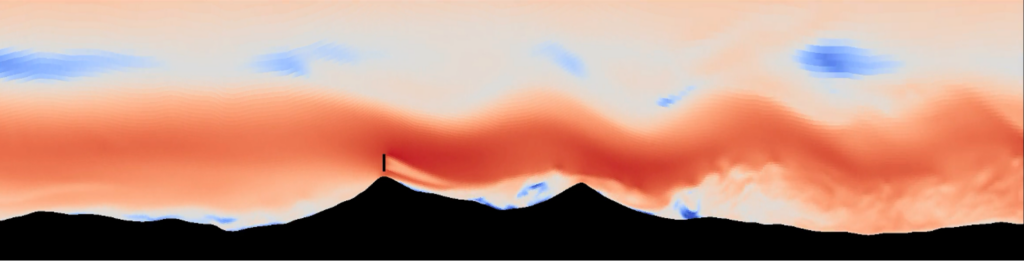
New turbulence closures for large-eddy simulation of clouds
Clouds are important to the earth’s energy balance and a regulator of both climate and weather. In global climate models, cloud feedbacks are a leading source of uncertainty. We are performing large-eddy simulations of clouds using the dynamic reconstruction turbulence model (DRM). We have found that the DRM enables simulations of clouds with coarser resolutions and less computation time without much compromise in accuracy (Shi et al. 2018a). In addition, we uncovered additional shortcomings of traditional closure models, in particular related to the common assumption of a constant Prandtl number for scalar diffusion (Shi et al. 2018b). The new turbulence closure models will boost numerical models’ capability in simulating clouds, helping to advance understanding and predictions of the evolution of clouds and their effects on weather and climate.
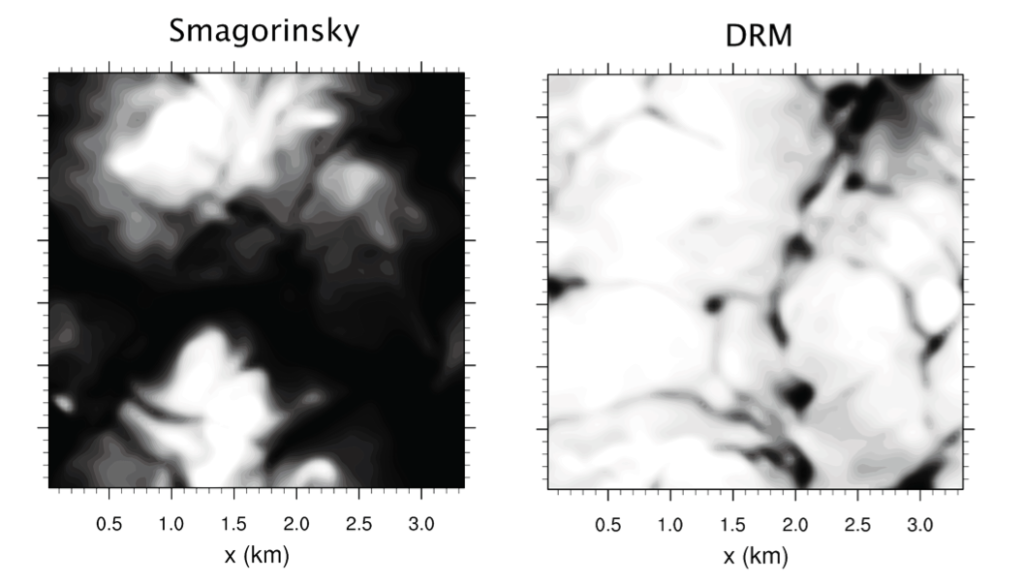
Large-eddy simulation for wind energy applications
Wind turbine micrositing, for operational wind power forecasting and for turbine design, requires high-resolution simulations of atmospheric flow over complex terrain. We are developing large-eddy simulation (LES) for wind energy applications using the Weather Research and Forecasting (WRF) model. Grid nesting is used to refine the grid from mesoscale to LES scales which can adequately resolve terrain and turbulence in the atmospheric boundary layer. Grid nesting provides time-dependent lateral boundary conditions which incorporate changing weather conditions even in the smallest domain. In an initial grid nesting study, the goal was to understand what model configurations are needed to predict rapid wind changes called “ramping” events. These ramping events are notoriously difficult for wind farm operators to predict, giving uncertainties in energy yield which can be quite costly. We found that as long as the topography is adequately resolved, higher model resolution was not necessary, and afforded very little improvement in model accuracy. This can help save computational resources when forecasting for a wind farm. We have also implemented an actuator line model into WRF to represent the individual blades of the turbine as they rotate, providing a very detailed representation of the wake of the turbine. See Marjanovic et al. 2014, Marjanovic et al. 2017 for details.
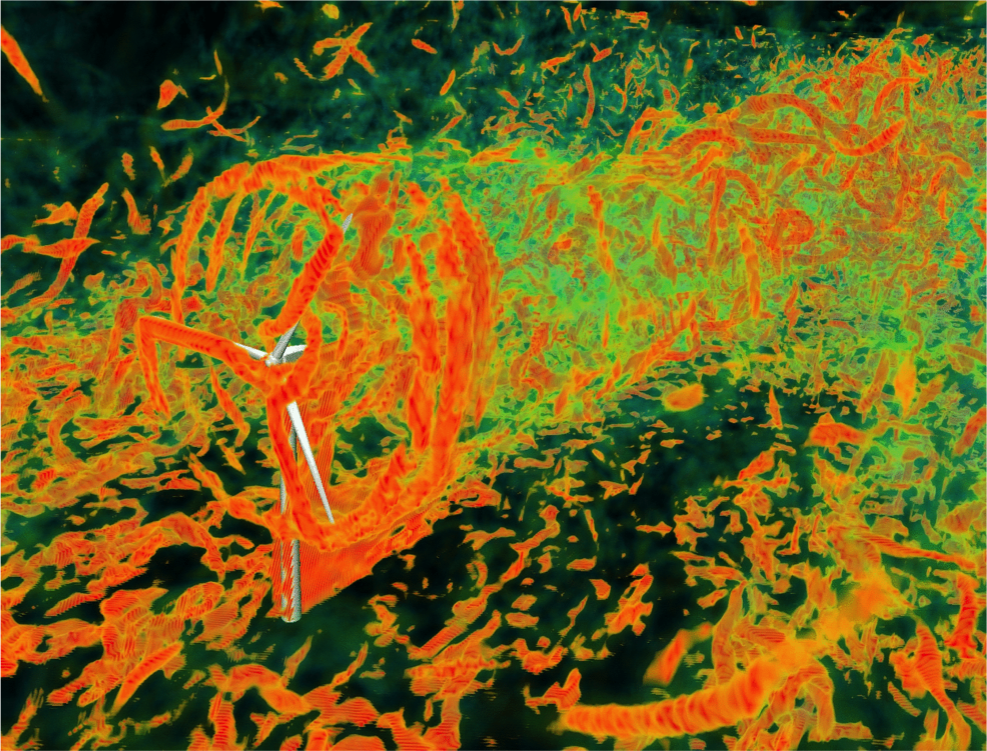
The immersed boundary method – urban dispersion modeling
A major difficulty in atmospheric modeling is the representation of the steep “terrain” associated with very steep mountains or vertical building surfaces. The problem is two-fold: first, current weather prediction models (such as WRF) are limited to modeling slopes of a maximum around 30 degrees, and second, engineering flow models which could resolve urban geometries (buildings) do not include the influence of external meteorological forcing. In collaboration with Katie Lundquist at LLNL, we are developing and implementing an immersed boundary method (IBM) into the Weather and Research Forecasting (WRF) model, which will enable simulation over steep terrain surfaces within an atmospheric model. IBM improves the representation of steep terrain and allows representation of vertical surfaces (like buildings). WRF can now be used at the urban scale, but to make IBM robust and generally applicable, it needs to be compatible with nesting within WRF, use rough wall boundary conditions, and allow vertical grid refinement. Each of these features is in progress and will enable urban and complex terrain flow studies in a fully nested environment using the most sophisticated atmospheric physics modules available in WRF. See Wiersema et al. 2020, 2022.
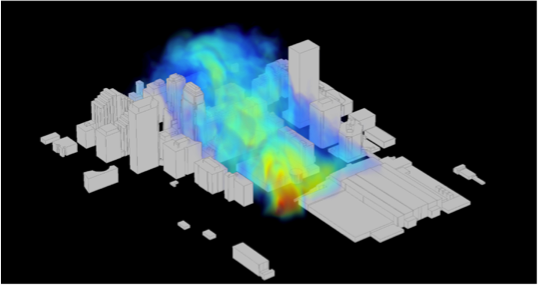
The MATERHORN project – flow over complex terrain
The Office of Naval Research funded MATERHORN project is a large collaborative effort led by the University Notre Dame with several partner institutions. A set of field campaigns at Dugway Proving Ground in Utah were completed in 2012-13 and an overview article describing the whole program is in the Bulletin of the AMS (Fernando et al. 2015). Our role is performing numerical simulations of stable slope flows on Granite Mountain. At the high resolutions needed to resolve these stable flows, the terrain steepness becomes such that traditional numerical models (such as the Weather and Research Forecasting (WRF) model, a community mesoscale model developed by NCAR) encounter numerical stability limits. Using the immersed boundary method (IBM) with WRF, we quantified the errors that result with increased terrain steepness and implemented topographic shading. See Bao et al. 2018, Arthur et al. 2018 and 2020.
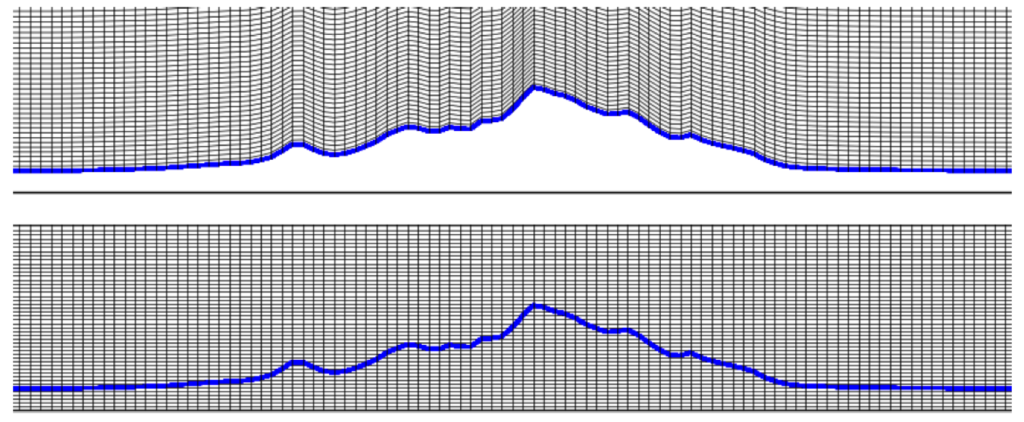
Explicit filtering and turbulence modeling for LES
The technique of large-eddy simulation (LES) resolves the largest turbulent motions in the atmosphere, but a turbulence closure model is needed to represent smaller scale motions. Under nighttime conditions, stable stratification occurs, such that turbulence is damped and often intermittent, making it hard for large-eddy simulation to faithfully represent fluid motions. Our explicit filtering and reconstruction turbulence model has the ability to simulate very strongly stable conditions in the atmosphere, where previous modeling attempts have failed (simulations could not sustain the surface cooling). The modeling results also confirm the presence of an “upside down” boundary layer structure that has been observed in field measurements of the stable boundary layer. This work first investigated idealized flows and then two real cases over complex terrain, one in the Great Plains (CASES-99 field data) and one in the Sierras (using T-REX field data) (see Zhou and Chow 2011, 2012, 2013, 2014).

Adaptive mesh refinement and LES – explicit filtering and turbulence modeling
Adaptive mesh refinement (AMR) is used to refine the grid near solid boundaries – for example, it is commonly used in urban dispersion simulations to refine the flow around buildings. LES and AMR cannot easily be used together because of underlying limitations in each approach, which lead to turbulent flow features in LES becoming distorted by grid refinement interfaces. We have performed idealized simulations of homogeneous turbulence with a grid refinement boundary to represent a simple AMR scenario and investigate the effect of the refinement interface on the development of turbulent structures in the flow. We found that use of our explicit filtering and reconstruction turbulence modeling framework helps to reduce numerical errors at the grid interface. See Goodfriend et al. 2013, 2015, 2016. This work is done in collaboration with Elias Balaras and Marcos Vanella at George Washington University.
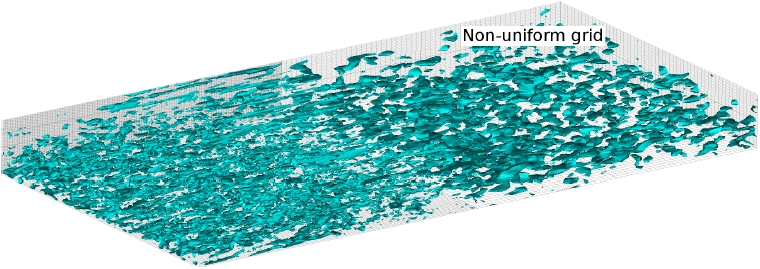
The Terrain-induced Rotor Experiment – Owens Valley
Owens Valley, California is famous for many reasons, from battles over its water resources (the Los Angeles Aqueduct starts here), to the “Sierra Wave” and the daring flights of the Sierra Wave Project in the 1950s. Owens Valley was the site of the Terrain-induced Rotor Experiment (T-REX) field campaign in Spring 2006, which focused on understanding lee waves and atmospheric rotors generated by strong winds over the Sierra-Nevada mountains. We performed high resolution simulations of nocturnal quiescent conditions in Owens Valley which explained peculiar physical phenomena which were observed in the field. For example, our simulations shed light on an unusual nighttime warming event (in contrast to the typically expected nighttime cooling). This warming event was found to be generated by a shear-induced mixing event which brought warm air from aloft down to the ground. The simulations also discovered a mechanism for intermittent turbulence observed during the stably-stratified nighttime period. A cold-air intrusion was observed on the eastern slope of the valley which generated a series of Kelvin-Helmholtz billows which propagated across the valley. The agreement with field data in this study was impressive because the intermittent nature of the turbulence was well captured in our model, which is typically difficult to do. See Zhou and Chow 2013, Schmidli et al. 2009, and Grubisic et al. 2008.
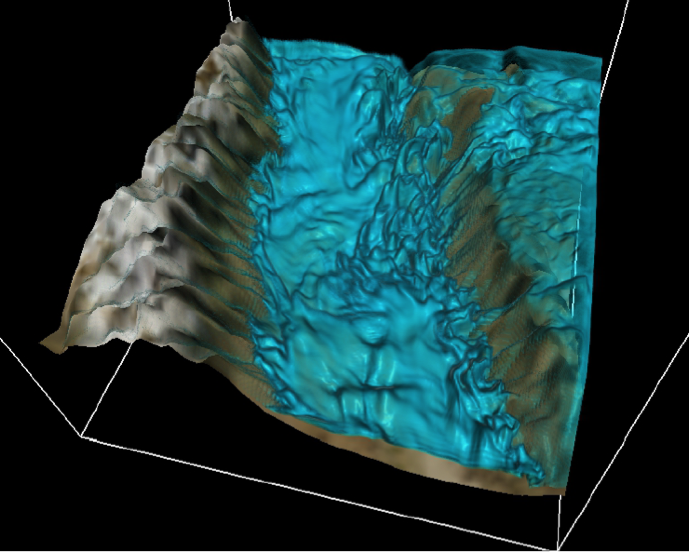